In Order for Chlorophyll to Absorb Light Again How Does It Replace the Lost Electron in Ps1 Psii
The Light-Dependent Reactions
Photosynthesis takes place in two stages: the light-dependent reactions and the Calvin cycle. In the light-dependent reactions, which take place at the thylakoid membrane, chlorophyll absorbs energy from sunlight and then converts it into chemical energy with the use of water. The light-dependent reactions release oxygen as a byproduct as water is broken apart. In the Calvin cycle, which takes place in the stroma, the chemical energy derived from the light-dependent reactions drives both the capture of carbon in carbon dioxide molecules and the subsequent assembly of sugar molecules.
The two reactions use carrier molecules to transport the energy from one to the other. The carriers that move energy from the light-dependent reactions to the Calvin cycle reactions can be thought of as "full" because they bring energy. After the energy is released, the "empty" energy carriers return to the light-dependent reactions to obtain more energy. You should be familiar with the energy carrier molecules used during cellular respiration: NADH and FADH2. Photosynthesis uses a different energy carrier, NADPH, but it functions in a comparable way. The lower energy form, NADP+, picks up a high energy electron and a proton and is converted to NADPH. When NADPH gives up its electron, it is converted back to NADP+.
How the Light-Dependent Reactions Work
The overall purpose of the light-dependent reactions is to convert solar energy into chemical energy in the form of NADPH and ATP. This chemical energy will be used by the Calvin cycle to fuel the assembly of sugar molecules.
The light-dependent reactions begin in a grouping of pigment molecules and proteins called a photosystem. There are two photosystems (Photosystem I and II), which exist in the membranes of thylakoids. Both photosystems have the same basic structure: a number of antenna proteins to which chlorophyll molecules are bound surround the reaction center where the photochemistry takes place. Each photosystem is serviced by the light-harvesting complex, which passes energy from sunlight to the reaction center. It consists of multiple antenna proteins that contain a mixture of 300–400 chlorophyll a and b molecules as well as other pigments like carotenoids. A photon of light energy travels until it reaches a molecule of chlorophyll pigment. The photon causes an electron in the chlorophyll to become "excited." The energy given to the electron allows it to break free from an atom of the chlorophyll molecule. Chlorophyll is therefore said to "donate" an electron (Figure 1).The absorption of a single photon or distinct quantity or "packet" of light by any of the chlorophylls pushes that molecule into an excited state. In short, the light energy has now been captured by biological molecules but is not stored in any useful form yet. The energy is transferred from chlorophyll to chlorophyll until eventually (after about a millionth of a second), it is delivered to the reaction center. Up to this point, only energy has been transferred between molecules, not electrons.
To replace the electron in the chlorophyll, a molecule of water is split. This splitting releases two electrons and results in the formation of oxygen (O2) and 2 hydrogen ions (H+) in the thylakoid space. The replacement of the electron enables chlorophyll to respond to another photon. The oxygen molecules produced as byproducts exit the leaf through the stomata and find their way to the surrounding environment. The hydrogen ions play critical roles in the remainder of the light-dependent reactions.
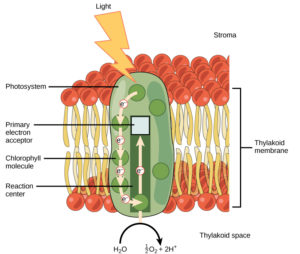
Keep in mind that the purpose of the light-dependent reactions is to convert solar energy into chemical carriers (NADPH and ATP) that will be used in the Calvin cycle. In eukaryotes and some prokaryotes, two photosystems exist. The first is called photosystem II(PSII), which was named for the order of its discovery rather than for the order of the function. After a photon hits the photosystem II (PSII) reaction center, energy from sunlight is used to extract electrons from water. The electrons travel through the chloroplast electron transport chain to photosystem I (PSI), which reduces NADP+ to NADPH (Figure 3). As the electron passes along the electron transport chain, energy from the electron fuels proton pumps in the membrane that actively move hydrogen ions against their concentration gradient from the stroma into the thylakoid space. The electron transport chain moves protons across the thylakoid membrane into the lumen (the space inside the thylakoid disk). At the same time, splitting of water adds additional protons into the lumen, and reduction of NADPH removes protons from the stroma (the space outside the thylakoids). The net result is a high concentration of protons (H+) in the thylakoid lumen, and a low concentration of protons in the stroma. ATP synthase uses this electrochemical gradient to make ATP, just like it did in cellular respiration. Note that a high concentration of protons = an acidic pH, so the thylakoid lumen has a much more acidic (lower) pH than the stroma.
This whole process is quite analogous to the process that occurs during cellular respiration in the mitochondria. Recall that during CR, the energy carried by NADH and FADH2 is used to pump protons across the inner mitochondrial membrane and into the intermembrane space, creating an electrochemical proton gradient. This gradient is used to power oxidative phosphorylation by ATP synthase to create ATP.
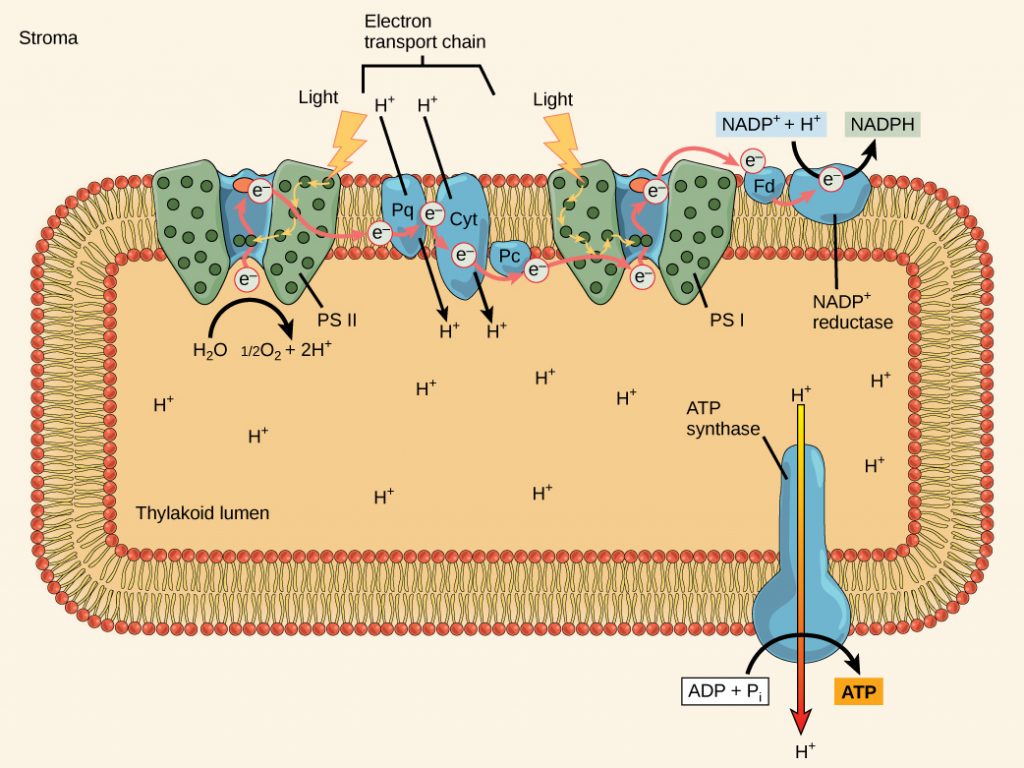
Generating an Energy Molecule: ATP
In the light-dependent reactions, energy absorbed by sunlight is stored by two types of energy-carrier molecules: ATP and NADPH. The energy that these molecules carry is stored in a bond that holds a single atom to the molecule. For ATP, it is a phosphate atom, and for NADPH, it is a hydrogen atom. Recall that NADH was a similar molecule that carried energy in the mitochondrion from the citric acid cycle to the electron transport chain. When these molecules release energy into the Calvin cycle, they each lose atoms to become the lower-energy molecules ADP and NADP+.
The buildup of hydrogen ions in the thylakoid space forms an electrochemical gradient because of the difference in the concentration of protons (H+) and the difference in the charge across the membrane that they create. This potential energy is harvested and stored as chemical energy in ATP through chemiosmosis, the movement of hydrogen ions down their electrochemical gradient through the transmembrane enzyme ATP synthase, just as in the mitochondrion.
The hydrogen ions are allowed to pass through the thylakoid membrane through an embedded protein complex called ATP synthase. This same protein generated ATP from ADP in the mitochondrion. The energy generated by the hydrogen ion stream allows ATP synthase to attach a third phosphate to ADP, which forms a molecule of ATP in a process called photophosphorylation. The flow of hydrogen ions through ATP synthase is called chemiosmosis (just like in cellular respiration), because the ions move from an area of high to low concentration through a semi-permeable structure.
Generating Another Energy Carrier: NADPH
The remaining function of the light-dependent reaction is to generate the other energy-carrier molecule, NADPH. As the electron from the electron transport chain arrives at photosystem I, it is re-energized with another photon captured by chlorophyll. The energy from this electron drives the formation of NADPH from NADP+ and a hydrogen ion (H+). Now that the solar energy is stored in energy carriers, it can be used to make a sugar molecule.
Section Summary
The pigments of the first part of photosynthesis, the light-dependent reactions, absorb energy from sunlight. A photon strikes the antenna pigments of photosystem II to initiate photosynthesis. The energy travels to the reaction center that contains chlorophyll a to the electron transport chain, which pumps hydrogen ions into the thylakoid interior (the lumen). This action builds up a high concentration of hydrogen ions. The ions flow through ATP synthase via chemiosmosis to form molecules of ATP, which are used for the formation of sugar molecules in the second stage of photosynthesis. Photosystem I absorbs a second photon, which results in the formation of an NADPH molecule, another energy and reducing power carrier for the light-independent reactions.
References
Unless otherwise noted, images on this page are licensed under CC-BY 4.0 by OpenStax.
Text adapted from: OpenStax, Concepts of Biology. OpenStax CNX. May 18, 2016 http://cnx.org/contents/b3c1e1d2-839c-42b0-a314-e119a8aafbdd@9.10
Source: https://openoregon.pressbooks.pub/mhccmajorsbio/chapter/8-3-the-two-parts-of-photosynthesis-light-dependent-reactions/
0 Response to "In Order for Chlorophyll to Absorb Light Again How Does It Replace the Lost Electron in Ps1 Psii"
Post a Comment